A new approach to analyse binary neutron star mergers, developed by Dr. Gregory Ashton (Royal Holloway, University of London) and Prof. Dr. Tim Dietrich (Potsdam University/Max Planck Institute for Gravitational Physics), was published in “Nature Astronomy” today. Their innovative technique provides a proving ground for model development and a means to identify systematics in future gravitational-wave observations.
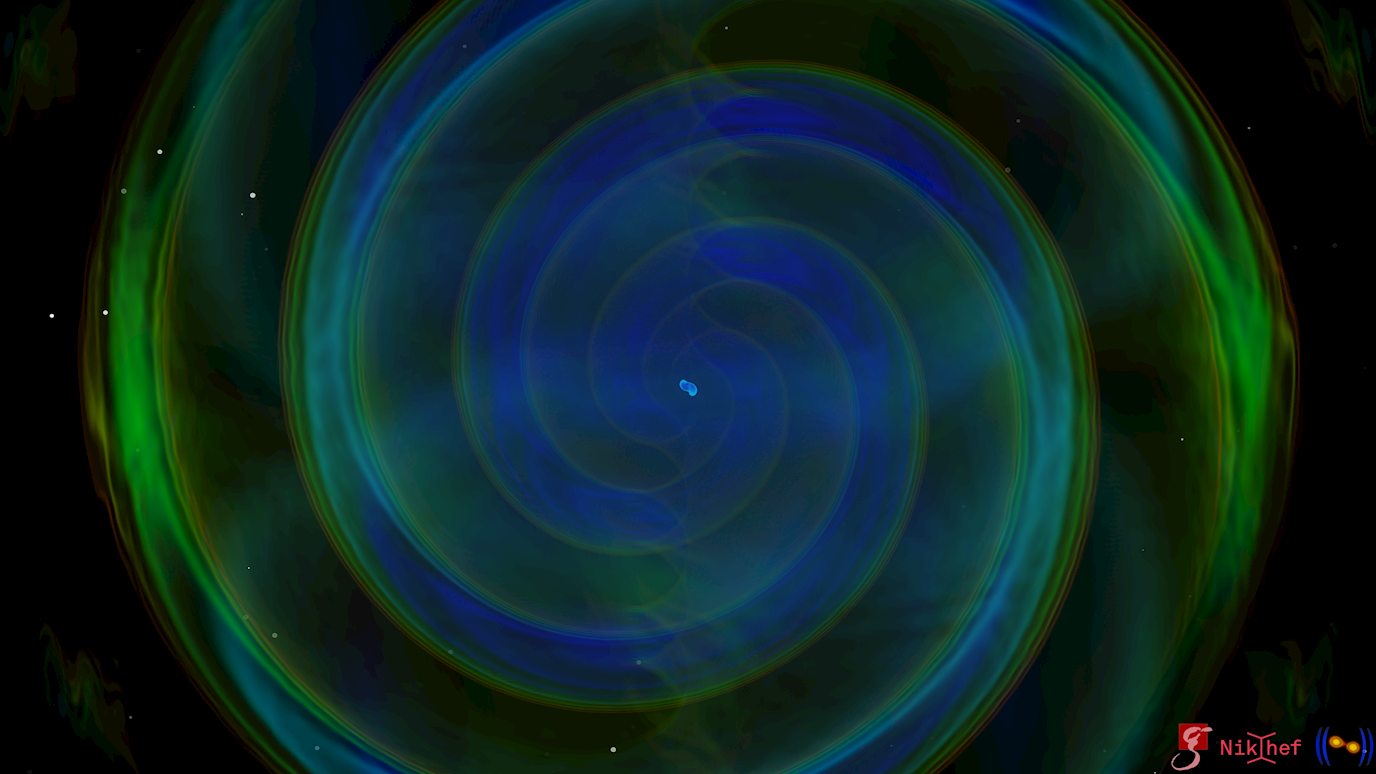
Numerical-relativity simulation of the binary neutron star coalescence and merger which resulted in the detected gravitational wave event GW190425. Image Credit: T. Dietrich (UP), S. Ossokine, A. Buonanno (Max Planck Institute for Gravitational Physics), W. Tichy (Florida Atlantic University) and the CoRe-collaboration.
At the end of the life of a massive star, an extremely dense neutron star can be born in a supernova explosion: one teaspoon of neutron star material would weight up to 1 billion tons. Given that neutron stars are so extremely compact, merging of two neutron stars represents a collision of unimaginable proportions. An enormous amount of energy gets released during the collision of such stars and gravitational waves, tiny ripples in the fabric of spacetimes, get emitted. Two well-observed neutron star merger events are GW170817 and GW190425. GW is short for “gravitational wave” and their name refers the date when these events have been observed. Both GW170817 and GW190425 were detected with the laser interferometers advanced LIGO and advanced Virgo here on Earth. In the case of GW170817 it was even possible to measure electromagnetic signals in the gamma-rays, X-rays, ultraviolet, optical, infrared, and radio bands.
Scientists try to model the final stages of coalescence and the gravitational-wave signal emitted with numerical-relativity simulations on high-performance computers. “The direct computation of the gravitational waves is a challenging task”, says Tim Dietrich, “because Einstein’s Field Equations, which govern the final stages of the collision, are extremely hard to solve.” Therefore, approximate models are used. “With our hypermodel approach we are able to tests gravitational-wave model assumptions without computationally expensive numerical-relativity simulations, but with the help of the observed gravitational-wave data”, explains Greg Ashton. The two scientists applied their approach to the two confidently detected binary neutron star collisions GW170817 and GW190425 and found a consistent preference for a specific waveform model that is subtly better at explaining the observed data. “Since gravitational-wave detectors will be more sensitive in the future thanks to developments in instrumentation, such subtle differences will become more prominent. Quantifying waveform-model systematics will then allow us to place tighter constraints on fundamental physics principles”, summarises Tim Dietrich.
The research article is available at Nature Astronomy.